
Thermonuclear weapon


Some designs use spherical secondaries.
- fission primary stage
- fusion secondary stage
- High-explosive lenses
- Uranium-238 ("tamper") lined with beryllium reflector
- Vacuum ("levitated core")
- Tritium "boost" gas (blue) within plutonium or uranium hollow core
- Radiation channel filled with polystyrene foam
- Uranium ("pusher/tamper")
- Lithium-6 deuteride (fusion fuel)
- Plutonium ("spark plug")
- Radiation case (confines thermal X-rays by reflection)
A thermonuclear weapon, fusion weapon or hydrogen bomb (H bomb) is a second-generation nuclear weapon design. Its greater sophistication affords it vastly greater destructive power than first-generation nuclear bombs, a more compact size, a lower mass, or a combination of these benefits. Characteristics of nuclear fusion reactions make possible the use of non-fissile depleted uranium as the weapon's main fuel, thus allowing more efficient use of scarce fissile material such as uranium-235 (235
U
) or plutonium-239 (239
Pu
). The first full-scale thermonuclear test (Ivy Mike) was carried out by the United States in 1952, and the concept has since been employed by most of the world's nuclear powers in the design of their weapons.[1]

Modern fusion weapons essentially consist of two main components: a nuclear fission primary stage (fueled by 235
U
or 239
Pu
) and a separate nuclear fusion secondary stage containing thermonuclear fuel: heavy isotopes of hydrogen (deuterium and tritium) as the pure element or in modern weapons lithium deuteride. For this reason, thermonuclear weapons are often colloquially called hydrogen bombs or H-bombs.[note 1]

A fusion explosion begins with the detonation of the fission primary stage. Its temperature soars past 100 million kelvin, causing it to glow intensely with thermal ("soft") X-rays. These X-rays flood the void (the "radiation channel" often filled with polystyrene foam) between the primary and secondary assemblies placed within an enclosure called a radiation case, which confines the X-ray energy and resists its outward pressure. The distance separating the two assemblies ensures that debris fragments from the fission primary (which move much more slowly than X-ray photons) cannot disassemble the secondary before the fusion explosion runs to completion.

The secondary fusion stage—consisting of outer pusher/tamper, fusion fuel filler and central plutonium spark plug—is imploded by the X-ray energy impinging on its pusher/ tamper. This compresses the entire secondary stage and drives up the density of the plutonium spark plug. The density of the plutonium fuel rises to such an extent that the spark plug is driven into a supercritical state, and it begins a nuclear fission chain reaction. The fission products of this chain reaction heat the highly compressed (and thus super dense) thermonuclear fuel surrounding the spark plug to around 300 million kelvin, igniting fusion reactions between fusion fuel nuclei. In modern weapons fueled by lithium deuteride, the fissioning plutonium spark plug also emits free neutrons that collide with lithium nuclei and supply the tritium component of the thermonuclear fuel.

The secondary's relatively massive tamper (which resists outward expansion as the explosion proceeds) also serves as a thermal barrier to keep the fusion fuel filler from becoming too hot, which would spoil the compression. If made of uranium, enriched uranium or plutonium, the tamper captures fast fusion neutrons and undergoes fission itself, increasing the overall explosive yield. Additionally, in most designs the radiation case is also constructed of a material that undergoes fission driven by fast thermonuclear neutrons. Such bombs are classified as two stage weapons. Fast fission of the tamper and radiation case is the main contribution to the total yield and is the dominant process that produces radioactive fission product fallout.[2][3]

Before Ivy Mike, Operation Greenhouse in 1951 was the first American nuclear test series to test principles that led to the development of thermonuclear weapons. Sufficient fission was achieved to boost the associated fusion device, and enough was learned to achieve a full-scale device within a year. The design of all modern thermonuclear weapons in the United States is known as the Teller–Ulam configuration for its two chief contributors, Edward Teller and Stanisław Ulam, who developed it in 1951[4] for the United States, with certain concepts developed with the contribution of physicist John von Neumann. Similar devices were developed by the Soviet Union, United Kingdom, France, China and India.[5] The thermonuclear Tsar Bomba was the most powerful bomb ever detonated.[6] As thermonuclear weapons represent the most efficient design for weapon energy yield in weapons with yields above 50 kilotons of TNT (210 TJ), virtually all the nuclear weapons of this size deployed by the five nuclear-weapon states under the Non-Proliferation Treaty today are thermonuclear weapons using the Teller–Ulam design.[7]

Nuclear weapons |
---|
![]() |
Background |
Nuclear-armed states |
|
Public knowledge concerning nuclear weapon design
This section needs additional citations for verification. (December 2015) |
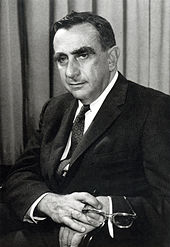
Detailed knowledge of fission and fusion weapons is classified to some degree in virtually every industrialized country. In the United States, such knowledge can by default be classified as "Restricted Data", even if it is created by persons who are not government employees or associated with weapons programs, in a legal doctrine known as "born secret" (though the constitutional standing of the doctrine has been at times called into question; see United States v. Progressive, Inc.). Born secret is rarely invoked for cases of private speculation. The official policy of the United States Department of Energy has been not to acknowledge the leaking of design information, as such acknowledgment would potentially validate the information as accurate. In a small number of prior cases, the U.S. government has attempted to censor weapons information in the public press, with limited success.[8] According to the New York Times, physicist Kenneth W. Ford defied government orders to remove classified information from his book Building the H Bomb: A Personal History. Ford claims he used only pre-existing information and even submitted a manuscript to the government, which wanted to remove entire sections of the book for concern that foreign states could use the information.[9]

Though large quantities of vague data have been officially released—and larger quantities of vague data have been unofficially leaked by former bomb designers—most public descriptions of nuclear weapon design details rely to some degree on speculation, reverse engineering from known information, or comparison with similar fields of physics (inertial confinement fusion is the primary example). Such processes have resulted in a body of unclassified knowledge about nuclear bombs that is generally consistent with official unclassified information releases and related physics and is thought to be internally consistent, though there are some points of interpretation that are still considered open. The state of public knowledge about the Teller–Ulam design has been mostly shaped from a few specific incidents outlined in a section below.

Basic principle
Primary and secondary stages
The basic principle of the Teller–Ulam configuration is the idea that different parts of a thermonuclear weapon can be chained together in stages, with the detonation of each stage providing the energy to ignite the next stage. At a minimum, this implies a primary section that consists of an implosion-type fission bomb (a "trigger"), and a secondary section that consists of fusion fuel. The energy released by the primary compresses the secondary through the process of radiation implosion, at which point it is heated and undergoes nuclear fusion. This process could be continued, with energy from the secondary igniting a third fusion stage; the Soviet Union's AN602 "Tsar Bomba" is thought to have been a three-stage fission-fusion-fusion device. Theoretically by continuing this process thermonuclear weapons with arbitrarily high yield could be constructed.[citation needed] This contrasts with fission weapons, which are limited in yield because only so much fission fuel can be amassed in one place before the danger of its accidentally becoming supercritical becomes too great.


Surrounding the other components is a hohlraum or radiation case, a container that traps the first stage or primary's energy inside temporarily. The outside of this radiation case, which is also normally the outside casing of the bomb, is the only direct visual evidence publicly available of any thermonuclear bomb component's configuration. Numerous photographs of various thermonuclear bomb exteriors have been declassified.[10]

The primary is thought[by whom?] to be a standard implosion method fission bomb, though likely with a core boosted by small amounts of fusion fuel (usually 1:1 deuterium:tritium gas) for extra efficiency; the fusion fuel releases excess neutrons when heated and compressed, inducing additional fission. When fired, the 239
Pu
or 235
U
core would be compressed to a smaller sphere by special layers of conventional high explosives arranged around it in an explosive lens pattern, initiating the nuclear chain reaction that powers the conventional "atomic bomb".
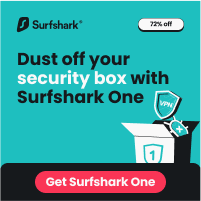
The secondary is usually shown as a column of fusion fuel and other components wrapped in many layers. Around the column is first a "pusher-tamper", a heavy layer of uranium-238 (238
U
) or lead that helps compress the fusion fuel (and, in the case of uranium, may eventually undergo fission itself). Inside this is the fusion fuel, usually a form of lithium deuteride, which is used because it is easier to weaponize than liquefied tritium/deuterium gas. This dry fuel, when bombarded by neutrons, produces tritium, a heavy isotope of hydrogen that can undergo nuclear fusion, along with the deuterium present in the mixture. (See the article on nuclear fusion for a more detailed technical discussion of fusion reactions.) Inside the layer of fuel is the "spark plug", a hollow column of fissile material (239
Pu
or 235
U
) often boosted by deuterium gas. The spark plug, when compressed, can undergo nuclear fission (because of the shape, it is not a critical mass without compression). The tertiary, if one is present, would be set below the secondary and probably be made of the same materials.[11][12]

Interstage
Separating the secondary from the primary is the interstage. The fissioning primary produces four types of energy: 1) expanding hot gases from high explosive charges that implode the primary; 2) superheated plasma that was originally the bomb's fissile material and its tamper; 3) the electromagnetic radiation; and 4) the neutrons from the primary's nuclear detonation. The interstage is responsible for accurately modulating the transfer of energy from the primary to the secondary. It must direct the hot gases, plasma, electromagnetic radiation and neutrons toward the right place at the right time. Less than optimal interstage designs have resulted in the secondary failing to work entirely on multiple shots, known as a "fissile fizzle". The Castle Koon shot of Operation Castle is a good example; a small flaw allowed the neutron flux from the primary to prematurely begin heating the secondary, weakening the compression enough to prevent any fusion.

There is very little detailed information in the open literature about the mechanism of the interstage. One of the best sources is a simplified diagram of a British thermonuclear weapon similar to the American W80 warhead. It was released by Greenpeace in a report titled "Dual Use Nuclear Technology".[13] The major components and their arrangement are in the diagram, though details are almost absent; what scattered details it does include likely have intentional omissions or inaccuracies. They are labeled "End-cap and Neutron Focus Lens" and "Reflector Wrap"; the former channels neutrons to the 235
U
/239
Pu
Spark Plug while the latter refers to an X-ray reflector; typically a cylinder made of an X-ray opaque material such as uranium with the primary and secondary at either end. It does not reflect like a mirror; instead, it gets heated to a high temperature by the X-ray flux from the primary, then it emits more evenly spread X-rays that travel to the secondary, causing what is known as radiation implosion. In Ivy Mike, gold was used as a coating over the uranium to enhance the blackbody effect.[14]

Next comes the "Reflector/Neutron Gun Carriage". The reflector seals the gap between the Neutron Focus Lens (in the center) and the outer casing near the primary. It separates the primary from the secondary and performs the same function as the previous reflector. There are about six neutron guns (seen here from Sandia National Laboratories[15]) each protruding through the outer edge of the reflector with one end in each section; all are clamped to the carriage and arranged more or less evenly around the casing's circumference. The neutron guns are tilted so the neutron emitting end of each gun end is pointed towards the central axis of the bomb. Neutrons from each neutron gun pass through and are focused by the neutron focus lens towards the centre of primary in order to boost the initial fissioning of the plutonium. A "polystyrene Polarizer/Plasma Source" is also shown (see below).

The first U.S. government document to mention the interstage was only recently released to the public promoting the 2004 initiation of the Reliable Replacement Warhead (RRW) Program. A graphic includes blurbs describing the potential advantage of a RRW on a part-by-part level, with the interstage blurb saying a new design would replace "toxic, brittle material" and "expensive 'special' material... [that require] unique facilities".[16] The "toxic, brittle material" is widely assumed to be beryllium, which fits that description and would also moderate the neutron flux from the primary. Some material to absorb and re-radiate the X-rays in a particular manner may also be used.[17]

Candidates for the "special material" are polystyrene and a substance called "Fogbank", an unclassified codename. Fogbank's composition is classified, though aerogel has been suggested as a possibility. It was first used in thermonuclear weapons with the W76 thermonuclear warhead and produced at a plant in the Y-12 Complex at Oak Ridge, Tennessee, for use in the W76. Production of Fogbank lapsed after the W76 production run ended. The W76 Life Extension Program required more Fogbank to be made. This was complicated by the fact that the original Fogbank's properties were not fully documented, so a massive effort was mounted to re-invent the process. An impurity crucial to the properties of the old Fogbank was omitted during the new process. Only close analysis of new and old batches revealed the nature of that impurity. The manufacturing process used acetonitrile as a solvent, which led to at least three evacuations of the Fogbank plant in 2006. Widely used in the petroleum and pharmaceutical industries, acetonitrile is flammable and toxic. Y-12 is the sole producer of Fogbank.[18]

Summary
A simplified summary of the above explanation is:

- A (relatively) small fission bomb known as the "primary" explodes.
- Energy released in the primary is transferred to the "secondary" (or fusion) stage. This energy compresses the fusion fuel and sparkplug; the compressed sparkplug becomes supercritical and undergoes a fission chain reaction, further heating the compressed fusion fuel to a high enough temperature to induce fusion.
- Energy released by the fusion events continues heating the fuel, keeping the reaction going.
- The fusion fuel of the secondary stage may be surrounded by a layer of additional fuel that undergoes fission when hit by the neutrons from the reactions within. These fission events account for about half of the total energy released in typical designs.
Compression of the secondary
How exactly the energy is "transported" from the primary to the secondary has been the subject of some disagreement in the open press but is thought to be transmitted through the X-rays and gamma rays that are emitted from the fissioning primary. This energy is then used to compress the secondary. The crucial detail of how the X-rays create the pressure is the main remaining disputed point in the unclassified press. There are three proposed theories:
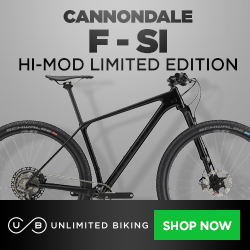
- Radiation pressure exerted by the X-rays. This was the first idea put forth by Howard Morland in an article in The Progressive.
- X-rays creating a plasma in the radiation channel's filler (a polystyrene or "Fogbank" plastic foam). This was a second idea put forward by Chuck Hansen and later by Howard Morland.
- Tamper/pusher ablation. This is the concept best supported by physical analysis.
Radiation pressure
The radiation pressure exerted by the large quantity of X-ray photons inside the closed casing might be enough to compress the secondary. Electromagnetic radiation such as X-rays or light carries momentum and exerts a force on any surface it strikes. The pressure of radiation at the intensities seen in everyday life, such as sunlight striking a surface, is usually imperceptible, but at the extreme intensities found in a thermonuclear bomb the pressure is enormous.

For two thermonuclear bombs for which the general size and primary characteristics are well understood, the Ivy Mike test bomb and the modern W-80 cruise missile warhead variant of the W-61 design, the radiation pressure was calculated to be 73×10 6 bar (7.3 TPa) for the Ivy Mike design and 1,400×10 6 bar (140 TPa) for the W-80.[19]

Foam plasma pressure
Foam plasma pressure is the concept that Chuck Hansen introduced during the Progressive case, based on research that located declassified documents listing special foams as liner components within the radiation case of thermonuclear weapons.
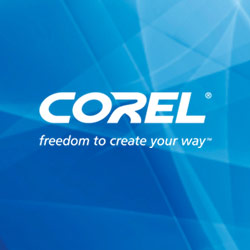
The sequence of firing the weapon (with the foam) would be as follows:

- The high explosives surrounding the core of the primary fire, compressing the fissile material into a supercritical state and beginning the fission chain reaction.
- The fissioning primary emits thermal X-rays, which "reflect" along the inside of the casing, irradiating the polystyrene foam.
- The irradiated foam becomes a hot plasma, pushing against the tamper of the secondary, compressing it tightly, and beginning the fission chain reaction in the spark plug.
- Pushed from both sides (from the primary and the spark plug), the lithium deuteride fuel is highly compressed and heated to thermonuclear temperatures. Also, by being bombarded with neutrons, each lithium-6 (6Li) atom splits into one tritium atom and one alpha particle. Then begins a fusion reaction between the tritium and the deuterium, releasing even more neutrons, and a huge amount of energy.
- The fuel undergoing the fusion reaction emits a large flux of high energy neutrons (17.6 MeV [2.82 pJ]), which irradiates the 238
U
tamper (or the 238
U
bomb casing), causing it to undergo a fast fission reaction, providing about half of the total energy.
This would complete the fission-fusion-fission sequence. Fusion, unlike fission, is relatively "clean"—it releases energy but no harmful radioactive products or large amounts of nuclear fallout. The fission reactions though, especially the last fission reactions, release a tremendous amount of fission products and fallout. If the last fission stage is omitted, by replacing the uranium tamper with one made of lead, for example, the overall explosive force is reduced by approximately half but the amount of fallout is relatively low. The neutron bomb is a hydrogen bomb with an intentionally thin tamper, allowing as many of the fast fusion neutrons as possible to escape.

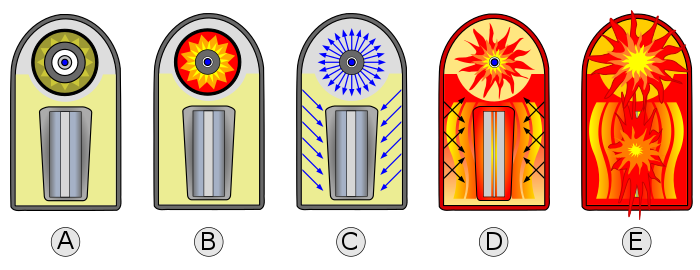
- Warhead before firing; primary (fission bomb) at top, secondary (fusion fuel) at bottom, all suspended in polystyrene foam.
- High-explosive fires in primary, compressing plutonium core into supercriticality and beginning a fission reaction.
- Fission primary emits X-rays that are scattered along the inside of the casing, irradiating the polystyrene foam.
- Polystyrene foam becomes plasma, compressing secondary, and plutonium sparkplug begins to fission.
- Compressed and heated, lithium-6 deuteride fuel produces tritium (3
H
) and begins the fusion reaction. The neutron flux produced causes the 238
U
tamper to fission. A fireball starts to form.
Current technical criticisms of the idea of "foam plasma pressure" focus on unclassified analysis from similar high energy physics fields that indicate that the pressure produced by such a plasma would only be a small multiplier of the basic photon pressure within the radiation case, and also that the known foam materials intrinsically have a very low absorption efficiency of the gamma ray and X-ray radiation from the primary. Most of the energy produced would be absorbed by either the walls of the radiation case or the tamper around the secondary. Analyzing the effects of that absorbed energy led to the third mechanism: ablation.

Tamper-pusher ablation
The outer casing of the secondary assembly is called the "tamper-pusher". The purpose of a tamper in an implosion bomb is to delay the expansion of the reacting fuel supply (which is very hot dense plasma) until the fuel is fully consumed and the explosion runs to completion. The same tamper material serves also as a pusher in that it is the medium by which the outside pressure (force acting on the surface area of the secondary) is transferred to the mass of fusion fuel.

The proposed tamper-pusher ablation mechanism posits that the outer layers of the thermonuclear secondary's tamper-pusher are heated so extremely by the primary's X-ray flux that they expand violently and ablate away (fly off). Because total momentum is conserved, this mass of high velocity ejecta impels the rest of the tamper-pusher to recoil inwards with tremendous force, crushing the fusion fuel and the spark plug. The tamper-pusher is built robustly enough to insulate the fusion fuel from the extreme heat outside; otherwise, the compression would be spoiled.
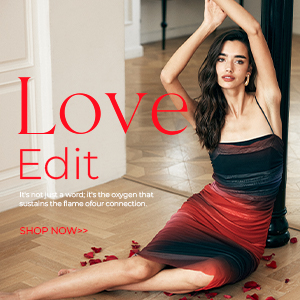

- Warhead before firing. The nested spheres at the top are the fission primary; the cylinders below are the fusion secondary device.
- Fission primary's explosives have detonated and collapsed the primary's fissile pit.
- The primary's fission reaction has run to completion, and the primary is now at several million degrees and radiating gamma and hard X-rays, heating up the inside of the hohlraum and the shield and secondary's tamper.
- The primary's reaction is over and it has expanded. The surface of the pusher for the secondary is now so hot that it is also ablating or expanding away, pushing the rest of the secondary (tamper, fusion fuel, and fissile spark plug) inwards. The spark plug starts to fission. Not depicted: the radiation case is also ablating and expanding outwards (omitted for clarity of diagram).
- The secondary's fuel has started the fusion reaction and shortly will burn up. A fireball starts to form.
Rough calculations for the basic ablation effect are relatively simple: the energy from the primary is distributed evenly onto all of the surfaces within the outer radiation case, with the components coming to a thermal equilibrium, and the effects of that thermal energy are then analyzed. The energy is mostly deposited within about one X-ray optical thickness of the tamper/pusher outer surface, and the temperature of that layer can then be calculated. The velocity at which the surface then expands outwards is calculated and, from a basic Newtonian momentum balance, the velocity at which the rest of the tamper implodes inwards.

Applying the more detailed form of those calculations to the Ivy Mike device yields vaporized pusher gas expansion velocity of 290 kilometres per second (29 cm/μs) and an implosion velocity of perhaps 400 km/s (40 cm/μs) if +3⁄4 of the total tamper/pusher mass is ablated off, the most energy efficient proportion. For the W-80 the gas expansion velocity is roughly 410 km/s (41 cm/μs) and the implosion velocity 570 km/s (57 cm/μs). The pressure due to the ablating material is calculated to be 5.3 billion bars (530 trillion pascals) in the Ivy Mike device and 64 billion bars (6.4 quadrillion pascals) in the W-80 device.[19]

Comparing implosion mechanisms
Comparing the three mechanisms proposed, it can be seen that:

Mechanism | Pressure (TPa) | |
---|---|---|
Ivy Mike | W80 | |
Radiation pressure | 7.3 | 140 |
Plasma pressure | 35 | 750 |
Ablation pressure | 530 | 6400 |
The calculated ablation pressure is one order of magnitude greater than the higher proposed plasma pressures and nearly two orders of magnitude greater than calculated radiation pressure. No mechanism to avoid the absorption of energy into the radiation case wall and the secondary tamper has been suggested, making ablation apparently unavoidable. The other mechanisms appear to be unneeded.

United States Department of Defense official declassification reports indicate that foamed plastic materials are or may be used in radiation case liners, and despite the low direct plasma pressure they may be of use in delaying the ablation until energy has distributed evenly and a sufficient fraction has reached the secondary's tamper/pusher.[20]

Richard Rhodes' book Dark Sun stated that a 1-inch-thick (25 mm) layer of plastic foam was fixed to the lead liner of the inside of the Ivy Mike steel casing using copper nails. Rhodes quotes several designers of that bomb explaining that the plastic foam layer inside the outer case is to delay ablation and thus recoil of the outer case: if the foam were not there, metal would ablate from the inside of the outer case with a large impulse, causing the casing to recoil outwards rapidly. The purpose of the casing is to contain the explosion for as long as possible, allowing as much X-ray ablation of the metallic surface of the secondary stage as possible, so it compresses the secondary efficiently, maximizing the fusion yield. Plastic foam has a low density, so causes a smaller impulse when it ablates than metal does.[20]

Design variations
Possible variations to the weapon design have been proposed:

- Either the tamper or the casing have been proposed to be made of 235
U
(highly enriched uranium) in the final fission jacket. The far more expensive 235
U
is also fissionable with fast neutrons like the 238
U
in depleted or natural uranium, but its fission-efficiency is higher. This is because 235
U
nuclei also undergo fission by slow neutrons (238
U
nuclei require a minimum energy of about 1 megaelectronvolt (0.16 pJ)) and because these slower neutrons are produced by other fissioning 235
U
nuclei in the jacket (in other words, 235
U
supports the nuclear chain reaction whereas 238
U
does not). Furthermore, a 235
U
jacket fosters neutron multiplication, whereas 238
U
nuclei consume fusion neutrons in the fast-fission process. Using a final fissionable/fissile jacket of 235
U
would thus increase the yield of a Teller–Ulam bomb above a depleted uranium or natural uranium jacket. This has been proposed specifically for the W87 warheads retrofitted to currently deployed LGM-30 Minuteman III ICBMs. - In some descriptions, additional internal structures exist to protect the secondary from receiving excessive neutrons from the primary.
- The inside of the casing may or may not be specially machined to "reflect" the X-rays. X-ray "reflection" is not like light reflecting off a mirror, but rather the reflector material is heated by the X-rays, causing the material to emit X-rays, which then travel to the secondary.
Most bombs do not apparently have tertiary "stages"—that is, third compression stage(s), which are additional fusion stages compressed by a previous fusion stage. The fissioning of the last blanket of uranium, which provides about half the yield in large bombs, does not count as a "stage" in this terminology.[citation needed]

The U.S. tested three-stage bombs in several explosions during Operation Redwing but is thought to have fielded only one such tertiary model, i.e., a bomb in which a fission stage, followed by a fusion stage, finally compresses yet another fusion stage. This U.S. design was the heavy but highly efficient (i.e., nuclear weapon yield per unit bomb weight) 25 Mt (100 PJ) B41 nuclear bomb.[21] The Soviet Union is thought to have used multiple stages (including more than one tertiary fusion stage) in their 50 Mt (210 PJ) (100 Mt (420 PJ) in intended use) Tsar Bomba. The fissionable jacket could be replaced with lead, as was done with the Tsar Bomba. If any hydrogen bombs have been made from configurations other than those based on the Teller–Ulam design, the fact of it is not publicly known. A possible exception to this is the Soviet early Sloika design.[citation needed]

In essence, the Teller–Ulam configuration relies on at least two instances of implosion occurring: first, the conventional (chemical) explosives in the primary would compress the fissile core, resulting in a fission explosion many times more powerful than that which chemical explosives could achieve alone (first stage). Second, the radiation from the fissioning of the primary would be used to compress and ignite the secondary fusion stage, resulting in a fusion explosion many times more powerful than the fission explosion alone. This chain of compression could conceivably be continued with an arbitrary number of tertiary fusion stages, each igniting more fusion fuel in the next stage[22]: 192–193 [23][better source needed] although this is debated. Finally, efficient bombs (but not so-called neutron bombs) end with the fissioning of the final natural uranium tamper, something that could not normally be achieved without the neutron flux provided by the fusion reactions in secondary or tertiary stages. Such designs are suggested to be capable of being scaled up to an arbitrary large yield (with apparently as many fusion stages as desired),[22]: 192–193 [23][better source needed] potentially to the level of a "doomsday device." However, usually such weapons were not more than a dozen megatons, which was generally considered enough to destroy even the most hardened practical targets (for example, a control facility such as the Cheyenne Mountain Complex). Even such large bombs have been replaced by smaller yield nuclear bunker buster bombs.[citation needed]

For destruction of cities and non-hardened targets, breaking the mass of a single missile payload down into smaller MIRV bombs in order to spread the energy of the explosions into a "pancake" area is far more efficient in terms of area-destruction per unit of bomb energy. This also applies to single bombs deliverable by cruise missile or other system, such as a bomber, resulting in most operational warheads in the U.S. program having yields of less than 500 kt (2,100 TJ).[citation needed]

Ivy Mike
In his 1995 book Dark Sun: The Making of the Hydrogen Bomb, author Richard Rhodes describes in detail the internal components of the "Ivy Mike" Sausage device, based on information obtained from extensive interviews with the scientists and engineers who assembled it. According to Rhodes, the actual mechanism for the compression of the secondary was a combination of the radiation pressure, foam plasma pressure, and tamper-pusher ablation theories; the radiation from the primary heated the polyethylene foam lining of the casing to a plasma, which then re-radiated radiation into the secondary's pusher, causing its surface to ablate and driving it inwards, compressing the secondary, igniting the sparkplug, and causing the fusion reaction. The general applicability of this principle is unclear.[14]

W88
In 1999 a reporter for the San Jose Mercury News reported that the U.S. W88 nuclear warhead, a small MIRVed warhead used on the Trident II SLBM, had a prolate primary (code-named Komodo) and a spherical secondary (code-named Cursa) inside a specially shaped radiation case (known as the "peanut" for its shape). The value of an egg-shaped primary lies apparently in the fact that a MIRV warhead is limited by the diameter of the primary: if an egg-shaped primary can be made to work properly, then the MIRV warhead can be made considerably smaller yet still deliver a high-yield explosion. A W88 warhead manages to yield up to 475 kilotonnes of TNT (1,990 TJ) with a physics package 68.9 inches (1,750 mm) long, with a maximum diameter of 21.8 inches (550 mm), and by different estimates weighing in a range from 175 to 360 kilograms (386 to 794 lb).[24] The smaller warhead allows more of them to fit onto a single missile and improves basic flight properties such as speed and range.[25]
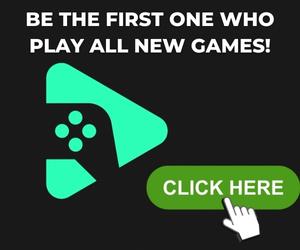
History
United States
The idea of a thermonuclear fusion bomb ignited by a smaller fission bomb was first proposed by Enrico Fermi to his colleague Edward Teller when they were talking at Columbia University in September 1941,[14]: 207 at the start of what would become the Manhattan Project.[4] Teller spent much of the Manhattan Project attempting to figure out how to make the design work, preferring it over work on the atomic bomb, and over the last year of the project he was assigned exclusively to the task.[14]: 117, 248 However once World War II ended, there was little impetus to devote many resources to the Super, as it was then known.[26]: 202 [27]

The first atomic bomb test by the Soviet Union in August 1949 came earlier than expected by Americans, and over the next several months there was an intense debate within the U.S. government, military, and scientific communities regarding whether to proceed with development of the far more powerful Super.[28]: 1–2 The debate covered matters that were alternatively strategic, pragmatic, and moral.[28]: 16 In their Report of the General Advisory Committee, Robert Oppenheimer and colleagues concluded that "[t]he extreme danger to mankind inherent in the proposal [to develop thermonuclear weapons] wholly outweighs any military advantage." Despite the objections raised, on 31 January 1950, President Harry S. Truman made the decision to go forward with the development of the new weapon.[26]: 212–214


Teller and other U.S. physicists struggled to find a workable design.[28]: 91–92 Stanislaw Ulam, a co-worker of Teller, made the first key conceptual leaps towards a workable fusion design. Ulam's two innovations that rendered the fusion bomb practical were that compression of the thermonuclear fuel before extreme heating was a practical path towards the conditions needed for fusion, and the idea of staging or placing a separate thermonuclear component outside a fission primary component, and somehow using the primary to compress the secondary. Teller then realized that the gamma and X-ray radiation produced in the primary could transfer enough energy into the secondary to create a successful implosion and fusion burn, if the whole assembly was wrapped in a hohlraum or radiation case.[4]

The "George" shot of Operation Greenhouse of 9 May 1951 tested the basic concept for the first time on a very small scale. As the first successful (uncontrolled) release of nuclear fusion energy, which made up a small fraction of the 225 kt (940 TJ) total yield,[29] it raised expectations to a near certainty that the concept would work. On 1 November 1952, the Teller–Ulam configuration was tested at full scale in the "Ivy Mike" shot at an island in the Enewetak Atoll, with a yield of 10.4 Mt (44 PJ) (over 450 times more powerful than the bomb dropped on Nagasaki during World War II). The device, dubbed the Sausage, used an extra-large fission bomb as a "trigger" and liquid deuterium—kept in its liquid state by 20 short tons (18 t) of cryogenic equipment—as its fusion fuel,[citation needed] and weighed around 80 short tons (73 t) altogether.

The liquid deuterium fuel of Ivy Mike was impractical for a deployable weapon, and the next advance was to use a solid lithium deuteride fusion fuel instead. In 1954 this was tested in the "Castle Bravo" shot (the device was code-named Shrimp), which had a yield of 15 Mt (63 PJ) (2.5 times expected) and is the largest U.S. bomb ever tested. Efforts shifted towards developing miniaturized Teller–Ulam weapons that could fit into intercontinental ballistic missiles and submarine-launched ballistic missiles. By 1960, with the W47 warhead[30] deployed on Polaris ballistic missile submarines, megaton-class warheads were as small as 18 inches (0.46 m) in diameter and 720 pounds (330 kg) in weight. Further innovation in miniaturizing warheads was accomplished by the mid-1970s, when versions of the Teller–Ulam design were created that could fit ten or more warheads on the end of a small MIRVed missile.[10]

Soviet Union
The first Soviet fusion design, developed by Andrei Sakharov and Vitaly Ginzburg in 1949 (before the Soviets had a working fission bomb), was dubbed the Sloika, after a Russian layer cake, and was not of the Teller–Ulam configuration. It used alternating layers of fissile material and lithium deuteride fusion fuel spiked with tritium (this was later dubbed Sakharov's "First Idea"). Though nuclear fusion might have been technically achievable, it did not have the scaling property of a "staged" weapon. Thus, such a design could not produce thermonuclear weapons whose explosive yields could be made arbitrarily large (unlike U.S. designs at that time). The fusion layer wrapped around the fission core could only moderately multiply the fission energy (modern Teller–Ulam designs can multiply it 30-fold). Additionally, the whole fusion stage had to be imploded by conventional explosives, along with the fission core, substantially increasing the amount of chemical explosives needed.

The first Sloika design test, RDS-6s, was detonated in 1953 with a yield equivalent to 400 kt (1,700 TJ) (15%-20% from fusion). Attempts to use a Sloika design to achieve megaton-range results proved unfeasible. After the United States tested the "Ivy Mike" thermonuclear device in November 1952, proving that a multimegaton bomb could be created, the Soviets searched for an alternative design. The "Second Idea", as Sakharov referred to it in his memoirs, was a previous proposal by Ginzburg in November 1948 to use lithium deuteride in the bomb, which would, in the course of being bombarded by neutrons, produce tritium and free deuterium.[31]: 299 In late 1953 physicist Viktor Davidenko achieved the first breakthrough of staging the reactions. The next breakthrough of radiation implosion was discovered and developed by Sakharov and Yakov Zel'dovich in early 1954. Sakharov's "Third Idea", as the Teller–Ulam design was known in the USSR, was tested in the shot "RDS-37" in November 1955 with a yield of 1.6 Mt (6.7 PJ). The Soviets demonstrated the power of the staging concept in October 1961, when they detonated the massive and unwieldy Tsar Bomba. It was the largest nuclear weapon developed and tested by any country.
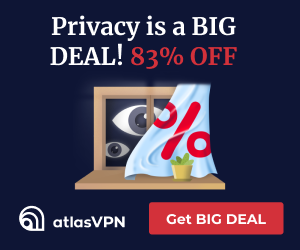
United Kingdom

In 1954 work began at Aldermaston to develop the British fusion bomb, with Sir William Penney in charge of the project. British knowledge on how to make a thermonuclear fusion bomb was rudimentary, and at the time the United States was not exchanging any nuclear knowledge because of the Atomic Energy Act of 1946. The United Kingdom had worked closely with the Americans on the Manhattan Project. British access to nuclear weapons information was cut off by the United States at one point due to concerns about Soviet espionage. Full cooperation was not reestablished until an agreement governing the handling of secret information and other issues was signed.[32][unreliable source?] However, the British were allowed to observe the U.S. Castle tests and used sampling aircraft in the mushroom clouds, providing them with clear, direct evidence of the compression produced in the secondary stages by radiation implosion.[32] Because of these difficulties, in 1955 Prime Minister Anthony Eden agreed to a secret plan, whereby if the Aldermaston scientists failed or were greatly delayed in developing the fusion bomb, it would be replaced by an extremely large fission bomb.[32]

In 1957 the Operation Grapple tests were carried out. The first test, Green Granite, was a prototype fusion bomb that failed to produce equivalent yields compared to the U.S. and Soviets, achieving only approximately 300 kt (1,300 TJ). The second test Orange Herald was the modified fission bomb and produced 720 kt (3,000 TJ)—making it the largest fission explosion ever. At the time almost everyone (including the pilots of the plane that dropped it) thought that this was a fusion bomb. This bomb was put into service in 1958. A second prototype fusion bomb, Purple Granite, was used in the third test, but only produced approximately 150 kt (630 TJ).[32]

A second set of tests was scheduled, with testing recommencing in September 1957. The first test was based on a "… new simpler design. A two-stage thermonuclear bomb that had a much more powerful trigger". This test Grapple X Round C was exploded on 8 November and yielded approximately 1.8 Mt (7.5 PJ). On 28 April 1958 a bomb was dropped that yielded 3 Mt (13 PJ)—Britain's most powerful test. Two final air burst tests on 2 and 11 September 1958, dropped smaller bombs that yielded around 1 Mt (4.2 PJ) each.[32]

American observers had been invited to these kinds of tests. After Britain's successful detonation of a megaton-range device (and thus demonstrating a practical understanding of the Teller–Ulam design "secret"), the United States agreed to exchange some of its nuclear designs with the United Kingdom, leading to the 1958 US–UK Mutual Defence Agreement. Instead of continuing with its own design, the British were given access to the design of the smaller American Mk 28 warhead and were able to manufacture copies.[32]

China
Mao Zedong decided to begin a Chinese nuclear-weapons program during the First Taiwan Strait Crisis of 1954–1955. The People's Republic of China detonated its first thermonuclear bomb on 17 June 1967, 32 months after detonating its first fission weapon, with a yield of 3.31 Mt. It took place in the Lop Nor Test Site in northwest China.[33] China had received extensive technical help from the Soviet Union to jump-start their nuclear program, but by 1960 the rift between the Soviet Union and China had become so great that the Soviet Union ceased all assistance to China.[34]

A story in The New York Times by William Broad[35] reported that in 1995, a supposed Chinese double agent delivered information indicating that China knew secret details of the U.S. W88 warhead, supposedly through espionage.[36] (This line of investigation eventually resulted in the abortive trial of Wen Ho Lee.)

France
The "Canopus" test in the Fangataufa atoll in French Polynesia on 24 August 1968 was the country's first multistage thermonuclear weapon test. The bomb was detonated from a balloon at a height of 520 metres (1,710 ft). The result of this test was significant atmospheric contamination.[37] Very little is known about France's development of the Teller–Ulam design, beyond the fact that France detonated a 2.6 Mt (11 PJ) device in the "Canopus" test. France reportedly had great difficulty with its initial development of the Teller-Ulam design, but it later overcame these and is believed to have nuclear weapons equal in sophistication to the other major nuclear powers.[32]

France and China did not sign or ratify the Partial Nuclear Test Ban Treaty of 1963, which banned nuclear test explosions in the atmosphere, underwater, or in outer space. Between 1966 and 1996 France carried out more than 190 nuclear tests.[37] France's final nuclear test took place on 27 January 1996, and then the country dismantled its Polynesian test sites. France signed the Comprehensive Nuclear-Test-Ban Treaty that same year, and then ratified the Treaty within two years.


In 2015 France confirmed that its nuclear arsenal contains about 300 warheads, carried by submarine-launched ballistic missiles and fighter-bombers. France has four Triomphant-class ballistic missile submarines. One ballistic missile submarine is deployed in the deep ocean, but a total of three must be in operational use at all times. The three older submarines are armed with 16 M45 missiles. The newest submarine, "Le Terrible", was commissioned in 2010, and it has M51 missiles capable of carrying TN 75 thermonuclear warheads. The air fleet is four squadrons at four different bases. In total, there are 23 Mirage 2000N aircraft and 20 Rafales capable of carrying nuclear warheads.[38] The M51.1 missiles are intended to be replaced with the new M51.2 warhead beginning in 2016, which has a 3,000 kilometres (1,900 mi) greater range than the M51.1.[38]

France has about 60 air-launched missiles tipped with TN 80/TN 81 warheads with a yield of about 300 kt (1,300 TJ) each. France's nuclear program has been carefully designed to ensure that these weapons remain usable decades into the future.[32][unreliable source?] Currently, France is no longer deliberately producing critical mass materials such as plutonium and enriched uranium, but it still relies on nuclear energy for electricity, with 239
Pu
as a byproduct.[39]

India

On 11 May 1998, India announced that it had detonated a thermonuclear bomb in its Operation Shakti tests ("Shakti-I", specifically, in Hindi the word 'Shakti' means power).[40][41] Samar Mubarakmand, a Pakistani nuclear physicist, asserted that if Shakti-I had been a thermonuclear test, the device had failed to fire.[42] However, Harold M. Agnew, former director of the Los Alamos National Laboratory, said that India's assertion of having detonated a staged thermonuclear bomb was believable.[5] India says that their thermonuclear device was tested at a controlled yield of 45 kt (190 TJ) because of the close proximity of the Khetolai village at about 5 kilometres (3.1 mi), to ensure that the houses in that village do not suffer significant damage.[43] Another cited reason was that radioactivity released from yields significantly more than 45 kt might not have been contained fully.[43] After the Pokhran-II tests, Rajagopala Chidambaram, former chairman of the Atomic Energy Commission of India, said that India has the capability to build thermonuclear bombs of any yield at will.[5] India officially maintains that it can build thermonuclear weapons of various yields up to around 200 kt (840 TJ) on the basis of the Shakti-1 thermonuclear test.[43][44]

The yield of India's hydrogen bomb test remains highly debatable among the Indian science community and the international scholars.[45] The question of politicisation and disputes between Indian scientists further complicated the matter.[46] In an interview in August 2009, the director for the 1998 test site preparations, K. Santhanam claimed that the yield of the thermonuclear explosion was lower than expected and that India should therefore not rush into signing the Comprehensive Nuclear-Test-Ban Treaty. Other Indian scientists involved in the test have disputed Santhanam's claim,[47] arguing that his claims are unscientific.[41] British seismologist Roger Clarke argued that the magnitudes suggested a combined yield of up to 60 kilotonnes of TNT (250 TJ), consistent with the Indian announced total yield of 56 kilotonnes of TNT (230 TJ).[48] U.S. seismologist Jack Evernden has argued that for correct estimation of yields, one should 'account properly for geological and seismological differences between test sites'.[43]
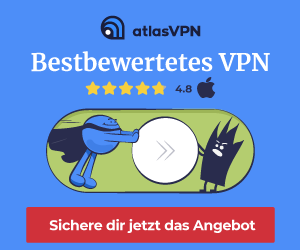
Israel
Israel is alleged to possess thermonuclear weapons of the Teller–Ulam design,[49] but it is not known to have tested any nuclear devices, although it is widely speculated that the Vela incident of 1979 may have been a joint Israeli–South African nuclear test.[50][51]: 271 [52]: 297–300

It is well established that Edward Teller advised and guided the Israeli establishment on general nuclear matters for some 20 years.[53]: 289–293 Between 1964 and 1967, Teller made six visits to Israel where he lectured at the Tel Aviv University on general topics in theoretical physics.[54] It took him a year to convince the CIA about Israel's capability and finally in 1976, Carl Duckett of the CIA testified to the U.S. Congress, after receiving credible information from an "American scientist" (Teller), on Israel's nuclear capability.[52]: 297–300 During the 1990s, Teller eventually confirmed speculations in the media that it was during his visits in the 1960s that he concluded that Israel was in possession of nuclear weapons.[52]: 297–300 After he conveyed the matter to the higher level of the U.S. government, Teller reportedly said: "They [Israel] have it, and they were clever enough to trust their research and not to test, they know that to test would get them into trouble."[52]: 297–300

North Korea
North Korea claimed to have tested its miniaturised thermonuclear bomb on 6 January 2016. North Korea's first three nuclear tests (2006, 2009 and 2013) were relatively low yield and do not appear to have been of a thermonuclear weapon design. In 2013, the South Korean Defense Ministry speculated that North Korea may be trying to develop a "hydrogen bomb" and such a device may be North Korea's next weapons test.[55][56] In January 2016, North Korea claimed to have successfully tested a hydrogen bomb,[57] although only a magnitude 5.1 seismic event was detected at the time of the test,[58] a similar magnitude to the 2013 test of a 6–9 kt (25–38 TJ) atomic bomb. These seismic recordings cast doubt upon North Korea's claim that a hydrogen bomb was tested and suggest it was a non-fusion nuclear test.[59]

On 3 September 2017, the country's state media reported that a hydrogen bomb test was conducted that resulted in "perfect success". According to the U.S. Geological Survey (USGS), the blast released energy equivalent to an earthquake with a seismic magnitude of 6.3, 10 times more powerful than previous nuclear tests conducted by North Korea.[60] U.S. Intelligence released an early assessment that the yield estimate was 140 kt (590 TJ),[61] with an uncertainty range of 70 to 280 kt (290 to 1,170 TJ).[62] On 12 September, NORSAR revised its estimate of the explosion magnitude upward to 6.1, matching that of the CTBTO but less powerful than the USGS estimate of 6.3. Its yield estimate was revised to 250 kt (1,000 TJ), while noting the estimate had some uncertainty and an undisclosed margin of error.[63][64] On 13 September, an analysis of before and after synthetic-aperture radar satellite imagery of the test site was published suggesting the test occurred under 900 metres (3,000 ft) of rock, and the yield "could have been in excess of 300 kilotons".[65]

Public knowledge
The Teller–Ulam design was for many years considered one of the top nuclear secrets, and even today it is not discussed in any detail by official publications with origins "behind the fence" of classification. United States Department of Energy (DOE) policy has been, and continues to be, that they do not acknowledge when "leaks" occur, because doing so would acknowledge the accuracy of the supposed leaked information. Aside from images of the warhead casing, most information in the public domain about this design is relegated to a few terse statements by the DOE and the work of a few individual investigators.

U.S. Department of Energy statements

In 1972 the United States government declassified a document stating "[I]n thermonuclear (TN) weapons, a fission 'primary' is used to trigger a TN reaction in thermonuclear fuel referred to as a 'secondary'", and in 1979 added, "[I]n thermonuclear weapons, radiation from a fission explosive can be contained and used to transfer energy to compress and ignite a physically separate component containing thermonuclear fuel." To this latter sentence the US government specified that "Any elaboration of this statement will be classified."[note 2] The only information that may pertain to the spark plug was declassified in 1991: "Fact that fissile or fissionable materials are present in some secondaries, material unidentified, location unspecified, use unspecified, and weapons undesignated." In 1998 the DOE declassified the statement that "The fact that materials may be present in channels and the term 'channel filler', with no elaboration", which may refer to the polystyrene foam (or an analogous substance).[66]

Whether these statements vindicate some or all of the models presented above is up for interpretation, and official U.S. government releases about the technical details of nuclear weapons have been purposely equivocating in the past (e.g., Smyth Report). Other information, such as the types of fuel used in some of the early weapons, has been declassified, though precise technical information has not been.

United States v. The Progressive
Most of the current ideas on the workings of the Teller–Ulam design came into public awareness after the DOE attempted to censor a magazine article by U.S. anti-weapons activist Howard Morland in 1979 on the "secret of the hydrogen bomb". In 1978, Morland had decided that discovering and exposing this "last remaining secret" would focus attention onto the arms race and allow citizens to feel empowered to question official statements on the importance of nuclear weapons and nuclear secrecy.[citation needed] Most of Morland's ideas about how the weapon worked were compiled from accessible sources: the drawings that most inspired his approach came from the Encyclopedia Americana.[citation needed] Morland also interviewed (often informally) many former Los Alamos scientists (including Teller and Ulam, though neither gave him any useful information), and he used a variety of interpersonal strategies to encourage informative responses from them (i.e., asking questions such as "Do they still use spark plugs?" even if he was not aware what the latter term specifically referred to).[67]

Morland eventually concluded that the "secret" was that the primary and secondary were kept separate and that radiation pressure from the primary compressed the secondary before igniting it. When an early draft of the article, to be published in The Progressive magazine, was sent to the DOE after falling into the hands of a professor who was opposed to Morland's goal, the DOE requested that the article not be published and pressed for a temporary injunction. The DOE argued that Morland's information was (1) likely derived from classified sources, (2) if not derived from classified sources, itself counted as "secret" information under the "born secret" clause of the 1954 Atomic Energy Act, and (3) was dangerous and would encourage nuclear proliferation. Morland and his lawyers disagreed on all points, but the injunction was granted, as the judge in the case felt that it was safer to grant the injunction and allow Morland, et al., to appeal.

Through a variety of more complicated circumstances, the DOE case began to wane as it became clear that some of the data they were attempting to claim as "secret" had been published in a students' encyclopedia a few years earlier. After another H-bomb speculator, Chuck Hansen, had his own ideas about the "secret" (quite different from Morland's) published in a Wisconsin newspaper, the DOE claimed that The Progressive case was moot, dropped its suit, and allowed the magazine to publish its article, which it did in November 1979. Morland had by then, however, changed his opinion of how the bomb worked, suggesting that a foam medium (the polystyrene) rather than radiation pressure was used to compress the secondary, and that in the secondary there was a spark plug of fissile material as well. He published these changes, based in part on the proceedings of the appeals trial, as a short erratum in The Progressive a month later.[68] In 1981, Morland published a book about his experience, describing in detail the train of thought that led him to his conclusions about the "secret".[67][69]

Morland's work is interpreted as being at least partially correct because the DOE had sought to censor it, one of the few times they violated their usual approach of not acknowledging "secret" material that had been released; however, to what degree it lacks information, or has incorrect information, is not known with any confidence. The difficulty that other countries had in developing the Teller–Ulam design (even when they apparently understood the design, such as with the United Kingdom) makes it somewhat unlikely that this simple information alone is what provides the ability to manufacture thermonuclear weapons. Nevertheless, the ideas put forward by Morland in 1979 have been the basis for all the current speculation on the Teller–Ulam design.

Notable accidents
On 5 February 1958, during a training mission flown by a B-47, a Mark 15 nuclear bomb, also known as the Tybee Bomb, was lost off the coast of Tybee Island near Savannah, Georgia. The US Air Force maintains that the bomb was unarmed and did not contain the live plutonium core necessary to initiate a nuclear explosion.[70] The bomb was thought by the Department of Energy to lie buried under several feet of silt at the bottom of Wassaw Sound.[71]

On 17 January 1966, a fatal collision occurred between a B-52G and a KC-135 Stratotanker over Palomares, Spain. The conventional explosives in two of the Mk28-type hydrogen bombs detonated upon impact with the ground, dispersing plutonium over nearby farms. A third bomb landed intact near Palomares while the fourth fell 12 miles (19 km) off the coast into the Mediterranean sea and was recovered a few months later.[72]

On 21 January 1968, a B-52G, with four B28FI thermonuclear bombs aboard as part of Operation Chrome Dome, crashed on the ice of the North Star Bay while attempting an emergency landing at Thule Air Base in Greenland.[73] The resulting fire caused extensive radioactive contamination.[74] Personnel involved in the cleanup failed to recover all the debris from three of the bombs, and one bomb was not recovered.[75]

See also
Notes
- ^ The misleading term hydrogen bomb was already in wide public use before fission product fallout from the Castle Bravo test in 1954 revealed the extent to which the design relies on fission as well.
- ^ emphasis in original
References
- ^ Conan, Neal (8 November 2005). "Sixty Years of Trying to Control the Bomb (Transcripts)". Talk of the Nation (Podcast). NPR. Retrieved 10 February 2021.
But, yes, the hydrogen bomb—that is, a two-stage thermonuclear device, as we refer to it—is indeed the principal part of the US arsenal, as it is of the Russian arsenal..
- ^ Gsponer, Andre (2005). Fourth Generation Nuclear Weapons: Military effectiveness and collateral effects. Independent Scientific Research Institute. arXiv:physics/0510071. ISRI-05-03.
- ^ Gsponer, Andre (2005). The B61-based "Robust Nuclear Earth Penetrator:" Clever retrofit or headway towards fourth-generation nuclear weapons?. Independent Scientific Research Institute. arXiv:physics/0510052. ISRI-03-08.
- ^ a b c Teller, Edward; Ulam, Stanislaw (9 March 1951). On Heterocatalytic Detonations I. Hydrodynamic Lenses and Radiation Mirrors (pdf) (Technical report). A. Los Alamos Scientific Laboratory. Archived (PDF) from the original on 28 November 2020. Retrieved 10 February 2021 – via Nuclear Non-Proliferation Institute.
- ^ a b c Burns, John F. (18 May 1998). "Nuclear Anxiety: The Overview; India Detonated a Hydrogen Bomb, Experts Confirm". The New York Times. ISSN 0362-4331. Retrieved 26 July 2019.
- ^ Magazine, Smithsonian; Machemer, Theresa. "Russia Declassifies Video From 1961 of Largest Hydrogen Bomb Ever Detonated". Smithsonian Magazine.
- ^ Sublette, Carey (3 July 2007). "Nuclear Weapons FAQ Section 4.4.1.4 The Teller–Ulam Design". Nuclear Weapons FAQ. Retrieved 17 July 2011. "So far as is known all high yield nuclear weapons today (>50 kt or so) use this design."
- ^ Broad, William J. (23 March 2015). "Hydrogen Bomb Physicist's Book Runs Afoul of Energy Department". The New York Times. Retrieved 20 November 2015.
- ^ Greene, Jes (25 March 2015). "A physicist might be in trouble for what he revealed in his new book about the H bomb". Business Insider. Retrieved 20 November 2015.
- ^ a b "Complete List of All U.S. Nuclear Weapons". 1 October 1997. Retrieved 13 March 2006.
- ^ Hansen, Chuck (1988). U.S. Nuclear Weapons: The Secret History. Crown. ISBN 978-0517567401. LCCN 87021995. OCLC 865554459. OL 2392513M. Retrieved 10 November 2021 – via Internet Archive.
- ^ Hansen, Chuck (2007). Swords of Armageddon: U.S. Nuclear Weapons Development Since 1945 (PDF) (CD-ROM & download available) (2nd ed.). Sunnyvale, California: Chukelea Publications. ISBN 978-0979191503.
- ^ "Figure 5 – Thermonuclear Warhead Components". Archived from the original on 12 July 2010. Retrieved 27 August 2010. A cleaned up version: "British H-bomb posted on the Internet by Greenpeace". Federation of American Scientists. Retrieved 27 August 2010.
- ^ a b c d Rhodes, Richard (1 August 1995). Dark Sun: The Making of the Hydrogen Bomb. Simon & Schuster. ISBN 978-0-68-480400-2. LCCN 95011070. OCLC 456652278. OL 7720934M. Wikidata Q105755363 – via Internet Archive.
- ^ Sublette, Carey (9 January 2007). "The W76 Warhead: Intermediate Yield Strategic SLBM MIRV Warhead". Nuclear Weapon Archive. Archived from the original on 26 January 2021. Retrieved 8 February 2021.
- ^ "Improved Security, Safety & Manufacturability of the Reliable Replacement Warhead" Archived 2008-12-17 at the Wayback Machine, NNSA March 2007.
- ^ A 1976 drawing that depicts an interstage that absorbs and re-radiates X-rays. From Howard Morland, "The Article", Cardozo Law Review, March 2005, p 1374.
- ^ Speculation on Fogbank, Arms Control Wonk
- ^ a b "Nuclear Weapons Frequently Asked Questions 4.4.3.3 The Ablation Process". 2.04. 20 February 1999. Retrieved 13 March 2006.
- ^ a b "Nuclear Weapons Frequently Asked Questions 4.4.4 Implosion Systems". 2.04. 20 February 1999. Retrieved 13 March 2006.
- ^ "The B-41 (Mk-41) Bomb – High yield strategic thermonuclear bomb". 21 October 1997. Retrieved 13 March 2006.
- ^ a b Winterberg, Friedwardt (2010). The Release of Thermonuclear Energy by Inertial Confinement: Ways Towards Ignition. World Scientific Publishing Company. ISBN 978-9814295901. OCLC 496957934. OL 26140529M – via Internet Archive.
- ^ a b Croddy, Eric A.; Wirtz, James J.; Larsen ear, Jeffrey A., eds. (2004). Weapons of Mass Destruction: An Encyclopedia of Worldwide Policy, Technology, and History. ABC-Clio. ISBN 978-1851094905. OCLC 941444356. OL 8969957M. Retrieved 8 February 2021 – via Google Books.
- ^ Harvey, John R.; Michalowski, Stefan (21 December 2007). "Nuclear weapons safety: The case of trident". Science & Global Security. 4 (1): 288. doi:10.1080/08929889408426405.
- ^ Stober, Dan; Hoffman, Ian (2001). A Convenient Spy: Wen Ho Lee and the Politics of Nuclear Espionage. Simon & Schuster. ISBN 978-0743223782. LCCN 2001054945. OL 7927314M – via Internet Archive.
- ^ a b Bundy, McGeorge (1988). Danger and Survival: Choices About the Bomb in the First Fifty Years. Random House. ISBN 978-0394522784. LCCN 89040089. OCLC 610771749. OL 24963545M.
- ^ Rhodes R (1995). Dark Sun: The Making of the Hydrogen Bomb. Simon & Schuster. ISBN 978-0-684-80400-2.
- ^ a b c Young, Ken; Schilling, Warner R. (2020). Super Bomb: Organizational Conflict and the Development of the Hydrogen Bomb. Cornell University Press. ISBN 978-1501745164. OCLC 1164620354. OL 28729278M.
- ^ "The 'George' shot, Comprehensive Test Ban Treaty Organisation website".
- ^ "Photograph of a W47 warhead" (JPG). Retrieved 13 March 2006.
- ^ Holloway, David (1994). Stalin and the Bomb: The Soviet Union and Atomic Energy, 1939–1956. Yale University Press. ISBN 978-0300060560. OCLC 470165274. OL 1084400M.
- ^ a b c d e f g h Younger, Stephen M. (2009). The Bomb: A New History. HarperCollins. ISBN 978-0061537196. OCLC 310470696. OL 24318509M – via Internet Archive].
- ^ "17 June 1967 – China's first thermonuclear test: CTBTO Preparatory Commission". www.ctbto.org. Retrieved 3 October 2016.
- ^ "China's Nuclear Weapon Development, Modernization and Testing". Nuclear Threat Initiative. 26 September 2003. Archived from the original on 8 October 2011. Retrieved 4 November 2011.
- ^ "Spies versus sweat, the debate over China's nuclear advance". The New York Times. 7 September 1999. Retrieved 18 April 2011.
- ^ Christopher Cox, chairman (1999). Report of the United States House of Representatives Select Committee on U.S. National Security and Military/Commercial Concerns with the People's Republic of China. Archived from the original on 4 August 2005., esp. Ch. 2, "PRC Theft of U.S. Thermonuclear Warhead Design Information".
- ^ a b "24 August 1968 – French 'Canopus' test: CTBTO Preparatory Commission". www.ctbto.org. Retrieved 15 April 2017.
- ^ a b "France | Countries | NTI". The Nuclear Threat Initiative. Retrieved 15 April 2017.
- ^ "Overview of the verification regime: CTBTO Preparatory Commission". www.ctbto.org. Retrieved 15 April 2017.
- ^ Burns, John F. (12 May 1998). "India Sets 3 Nuclear Blasts, Defying a Worldwide Ban; Tests Bring a Sharp Outcry". The New York Times. ISSN 0362-4331. Retrieved 24 December 2019.
- ^ a b "Pokhran – II tests were fully successful; given India capability to build nuclear deterrence: Dr. Kakodkar and Dr. Chidambaram". pib.nic.in. Retrieved 26 July 2019.
- ^ Sublette, Carey (10 September 2001). "Pakistan's Nuclear Weapons Program - 1998: The Year of Testing". The Nuclear Weapon Archive. Archived from the original on 10 August 2011. Retrieved 10 August 2011.
A. Q. Khan...said..."none of these explosions were thermonuclear, we are doing research and can do a fusion test if asked..." ""These boosted devices are like a half way stage towards a thermonuclear bomb. They use elements of the thermonuclear process, and are effectively stronger Atom bombs," Munir Ahmad Khan
- ^ a b c d "Press Statement by Dr. Anil Kakodkar and Dr. R. Chidambaram on Pokhran-II tests". Press Information Bureau. 24 September 2009. Archived from the original on 24 October 2017.
- ^ "Nukes of 200kt yield possible: Architect of Pokhran-II". The Times of India. 25 September 2009.
- ^ PTI, Press Trust of India (25 September 2009). "AEC ex-chief backs Santhanam on Pokhran-II". The Hindu, 2009. Retrieved 18 January 2013.
- ^ Sublette, Carey; et al. "What are the real yield of India's Test?". What Are the Real Yields of India's Test?. Retrieved 18 January 2013.
- ^ "Former NSA disagrees with scientist, says Pokhran II successful". The Times of India. 27 August 2009. Archived from the original on 30 August 2009. Retrieved 20 November 2015.
- ^ "We have an adequate scientific database for designing ... a credible nuclear deterrent". Frontline. 2 January 1999.
- ^ Samdani, Zafar (25 March 2000). "India, Pakistan can build hydrogen bomb: Scientist". Dawn News Interviews. Retrieved 23 December 2012.
- ^ "Doctrine", Israel, FAS.
- ^ Hersh, Seymour M. (1991). The Samson Option: Israel's Nuclear Arsenal & American Foreign Policy. New York: Random House. ISBN 978-0394570068. LCCN 91052678. OCLC 1159416022. OL 1567229M – via Internet Archive.
- ^ a b c d Cohen, Avner (1998). "Chapter 16: The Battle over the NPT". Israel and the Bomb. Columbia University Press (published 1999). ISBN 978-0585041506. LCCN 98003402. OCLC 42330721. OL 344440M – via Internet Archive.
- ^ Karpin, Michael I. (2006). The Bomb in the Basement: How Israel Went Nuclear and What That Means for the World. Simon & Schuster. ISBN 978-0743265942. LCCN 2005051689. OCLC 892937053. OL 3427490M – via Internet Archive.
- ^ Gábor Palló (2000). "The Hungarian Phenomenon in Israeli Science". Hungarian Academy of Science. Retrieved 11 December 2012.
- ^ Kim Kyu-won (7 February 2013). "North Korea could be developing a hydrogen bomb". The Hankyoreh. Retrieved 8 February 2013.
- ^ Kang Seung-woo; Chung Min-uck (4 February 2013). "North Korea may detonate H-bomb". Korea Times. Retrieved 8 February 2013.
- ^ "North Korea nuclear: State claims first hydrogen bomb test". BBC News. 6 January 2016.
- ^ M5.1 – 21 km ENE of Sungjibaegam, North Korea (Report). USGS. 6 January 2016. Retrieved 6 January 2016.
- ^ "North Korea nuclear H-bomb claims met by scepticism". BBC News. 6 January 2016.
- ^ "North Korea says conducts 'perfect' hydrogen bomb test". Reuters. 3 September 2017. Retrieved 10 September 2023.
- ^ Panda, Ankit (6 September 2017). "US Intelligence: North Korea's Sixth Test Was a 140 Kiloton 'Advanced Nuclear' Device". The Diplomat. Retrieved 6 September 2017.
- ^ Michelle Ye Hee Lee (13 September 2017). "North Korea nuclear test may have been twice as strong as first thought". Washington Post. Retrieved 28 September 2017.
- ^ "The nuclear explosion in North Korea on 3 September 2017: A revised magnitude assessment – NORSAR". Archived from the original on 13 September 2017. Retrieved 18 November 2017.
- ^ "North Korea's Punggye-ri Nuclear Test Site: Satellite Imagery Shows Post-Test Effects and New Activity in Alternate Tunnel Portal Areas | 38 North: Informed Analysis of North Korea". 12 September 2017.
- ^ Lewis, Jeffrey (13 September 2017). "SAR Image of Punggye-ri". Arms Control Wonk.
- ^ Restricted Data Declassification Decisions, 1946 to the present. Vol. 7. United States Department of Energy. January 2001.
- ^ a b Morland, Howard (1981). The secret that exploded. Random House. ISBN 978-0394512976. LCCN 80006032. OCLC 7196781. OL 4094494M.
- ^ "The H-Bomb Secret: How we got it and why we're telling it". The Progressive. 43 (11). November 1979.
- ^ De Volpi, Alexander; Marsh, Gerald E.; Postol, Ted; Stanford, George (1981). Born Secret: The H-Bomb, the Progressive Case and National Security. Pergamon Press. ISBN 978-0080259956. OCLC 558172005. OL 7311029M – via Internet Archive.
- ^ "Air Force Search & Recovery Assessment of the 1958 Savannah, GA B-47 Accident". AF Nuclear Weapons and Counterproliferation Agency. U.S. Air Force. 2001.
- ^ "For 50 Years, Nuclear Bomb Lost in Watery Grave". NPR. 3 February 2008.
- ^ "US to clean up Spanish radioactive site 49 years after plane crash". The Guardian. 19 October 2015.
- ^ "The Cold War's Missing Atom Bombs". Der Spiegel. 14 November 2008.
- ^ "US B-52 nuclear bomber crash in Greenland 51 years ago has ill Danes seeking compensation". Fox News. 3 June 2019.
- ^ Swaine, Jon (11 November 2008). "US left nuclear weapon under ice in Greenland". The Daily Telegraph. ISSN 0307-1235. OCLC 49632006. Archived from the original on 1 June 2009. Retrieved 10 February 2021.
Following the crash, and a painstaking clear-up mission to recover thousands of pieces of material from 500 million gallons of ice, the Pentagon stated that all four weapons onboard had been "destroyed". However, documents obtained by the BBC under the US Freedom of Information act have disclosed that while this is technically true—none of the bombs remained complete—one of the weapons was not recovered.
Further reading
Basic principles
- Sublette, Carey (19 March 2019). "Section 4.0 Engineering and Design of Nuclear Weapons". Nuclear Weapon Archive. Archived from the original on 6 February 2021. Retrieved 9 February 2021.
- Barroso, Dalton E. G. (2009). A Fisica dos Explosivos Nucleares [The Physics of Nuclear Explosives] (in Portuguese) (2nd ed.). Livraria da Física. ISBN 978-8578610166. OCLC 733273749. OL 30689359M. Retrieved 9 February 2020 – via Google Books.
History
- Bundy, McGeorge (1988). Danger and Survival: Choices About the Bomb in the First Fifty Years. Random House. ISBN 978-0394522784. LCCN 89040089. OCLC 610771749. OL 24963545M.
- DeGroot, Gerard J. (2005). The Bomb: A Life. Harvard University Press. ISBN 978-0674017245. OCLC 57750742. OL 7671320M – via Internet Archive.
- Galison, Peter; Bernstein, Barton J. (1 January 1989). "In Any Light: Scientists and the Decision to Build the Superbomb, 1952–1954". Historical Studies in the Physical and Biological Sciences. 19 (2): 267–347. doi:10.2307/27757627. eISSN 1939-182X. ISSN 1939-1811. JSTOR 27757627.
- Goncharov, German A. (31 October 1996). "American and Soviet H-bomb development programmes: historical background". Physics-Uspekhi. 39 (10): 1033–1044. Bibcode:1996PhyU...39.1033G. doi:10.1070/PU1996v039n10ABEH000174. eISSN 1468-4780. ISSN 1063-7869. LCCN 93646146. OCLC 36334507. S2CID 250861572.
- Holloway, David (1994). Stalin and the Bomb: The Soviet Union and Atomic Energy, 1939–1956. Yale University Press. ISBN 978-0300060560. OCLC 470165274. OL 1084400M.
- Rhodes, Richard (1995). Dark Sun: The Making of the Hydrogen Bomb. New York: Simon & Schuster. ISBN 978-0684804002. OCLC 717414304. OL 7720934M – via Internet Archive.
- Schweber, Silvan S. (2007). In the Shadow of the Bomb: Oppenheimer, Bethe, and the Moral Responsibility of the Scientist. Princeton Series in Physics. Princeton University Press. ISBN 978-0691127859. OCLC 868971191. OL 7757230M – via Internet Archive.
- Stix, Gary (20 October 1999). "Infamy and Honor at the Atomic Café: Father of the hydrogen bomb, "Star Wars" missile defense and Lawrence Livermore National Laboratory, Edward Teller has no regrets about his contentious career". Scientific American. Vol. 281, no. 4. pp. 42–43. ISSN 0036-8733.
- Young, Ken; Schilling, Warner R. (2020). Super Bomb: Organizational Conflict and the Development of the Hydrogen Bomb. Cornell University Press. ISBN 978-1501745164. OCLC 1164620354. OL 28729278M.
- Younger, Stephen M. (2009). The Bomb: A New History. HarperCollins. ISBN 978-0061537196. OCLC 310470696. OL 24318509M – via Internet Archive.
Analyzing fallout
- De Geer, Lars-Erik (1991). "The radioactive signature of the hydrogen bomb". Science & Global Security. 2 (4): 351–363. Bibcode:1991S&GS....2..351D. doi:10.1080/08929889108426372. ISSN 0892-9882. OCLC 15307789.
- Khariton, Yuli; Smirnov, Yuri; Rothstein, Linda; Leskov, Sergei (1993). "The Khariton Version". Bulletin of the Atomic Scientists. 49 (4): 20–31. Bibcode:1993BuAtS..49d..20K. doi:10.1080/00963402.1993.11456341. eISSN 1938-3282. ISSN 0096-3402. LCCN 48034039. OCLC 470268256.
External links
Principles
- "Basic Principles of Staged Radiation Implosion (Teller–Ulam)" from Carey Sublette's NuclearWeaponArchive.org.
- "Matter, Energy, and Radiation Hydrodynamics" from Carey Sublette's Nuclear Weapons FAQ.
- "Engineering and Design of Nuclear Weapons" from Carey Sublette's Nuclear Weapons FAQ.
- "Elements of Thermonuclear Weapon Design" from Carey Sublette's Nuclear Weapons FAQ.
- Annotated bibliography for nuclear weapons design from the Alsos Digital Library for Nuclear Issues
History
- PBS: Race for the Superbomb: Interviews and Transcripts Archived 11 March 2017 at the Wayback Machine (with U.S. and USSR bomb designers as well as historians).
- Howard Morland on how he discovered the "H-bomb secret" (includes many slides).
- The Progressive November 1979 issue – "The H-Bomb Secret: How we got it, why we're telling" (entire issue online).
- Annotated bibliography on the hydrogen bomb from the Alsos Digital Library
- University of Southampton, Mountbatten Centre for International Studies, Nuclear History Working Paper No5.
- Peter Kuran's "Trinity and Beyond" Archived 9 December 2018 at the Wayback Machine – documentary film on the history of nuclear weapon testing.
- YouTube Playlist of declassified nuclear explosion tests obtained by converting film scans to digital at Lawrence Livermore Laboratory
See what we do next...
OR
By submitting your email or phone number, you're giving mschf permission to send you email and/or recurring marketing texts. Data rates may apply. Text stop to cancel, help for help.
Success: You're subscribed now !